University Showcase Program (CuTS)
EDM3 Collaboration - Electron Electric Dipole Moment Experiments
York University
The Standard Model of particle physics is the most accurate mathematical description of reality ever constructed. It is, however, incomplete. Among other shortcomings, it sheds no light on the nature of the ‘dark matter’ that permeates galaxies and intergalactic space, did not predict that a group of fundamental particles known as neutrinos have mass, and cannot explain why the Big Bang so strongly favoured matter over antimatter when creating the contents of our universe. To account for these unexplained phenomena, various standard model ‘extensions’ have been formulated that propose the existence of new forces of nature manifested by as-yet undiscovered particles. Predictions of new particles have historically been tested with multi-billion-dollar particle accelerators like the Large Hadron Collider (or ‘LHC’), currently the largest machine on the face of the Earth. However, the LHC has not yet observed any fundamental particles other than those described by the current form of the SM.
Hope may lie in the prediction that electrons possess an uneven distribution of charge, the degree of unevenness being quantified by a number called the electric dipole moment (or ‘EDM’). Since each standard model extension predicts a different range of possible values for the electron’s EDM, measuring this quantity could verify a standard model extension – the holy grail of modern physics – and transform humanity’s understanding of the universe. Unfortunately, all experiments thus far have failed to detect a nonzero electron EDM.
Our experiment uses the fact that electrons in atoms and molecules will oscillate between different quantum states when put in a magnetic field, analogous to how a gyroscope will ‘precess’ in a circle when torqued by Earth’s gravity. We will use lasers and radio-frequency (or ‘RF) pulses to put a large population of electrons into two different states in which they will precess in opposite directions when an external magnetic field is applied. If electrons have an EDM then they will slowly precess in an applied electric field as well, which will cause a slight asymmetry in the total precession rate between the two states. This asymmetry will be detected using light emitted by the electrons after further laser and RF pulses. Rather than use a lab-generated electric field, we will exploit the enormous naturally-occurring electric field felt by electrons inside polar molecules by conducting our experiments on a population of barium monofluoride (or ‘BaF’) molecules. Due to the extreme reactivity of these molecules, we will trap them in a crystal of solid argon cooled to 4 kelvin (-269 degrees celsius) for the duration of the experiment. If successful, the immense precision promised by this tabletop experimental design would provide a model-independent test of fundamental physics at energy scales thousands of times higher than those reachable by the LHC.
The P50-502 and -505 thermal straps provided by TAI are instrumental in the operation of our apparatus by allowing certain critical components to reach 4 kelvin while also keeping them isolated from vibrations. These components form the source of our molecules, in which a pulsed laser is used to ablate a barium rod in the presence of sulphur hexafluoride gas inside of a cryogenic box filled with helium at 4 kelvin. The helium is used to rapidly thermalize the molecules before they exit the box through a hole into ultra high vacuum as a beam. However, the helium also exits through this hole and must be pumped away. This is achieved by cryosorbing the helium onto panels containing a porous material (coconut charcoal) at 4 kelvin - a process that becomes exponentially less efficient with even a few tenths of a degree increase in temperature.
In summary, just as a musician strums a harp to make it emit sound with properties determined by the harp’s tuning and architecture, we will ‘strum’ this BaF-doped argon crystal with precision lasers and RF pulses to make it emit light with properties determined by the fundamental architecture of the cosmos.
To learn more about the EDM3 Collaboration, visit our website here: http://www.yorku.ca/edmcubed/
- Neil Torrey McCall
Graduate Student
EDM-Cubed Collaboration
Department of Physics and Astronomy
The Majorana Demonstrator
University of North Carolina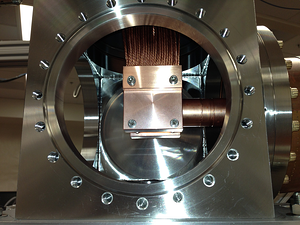
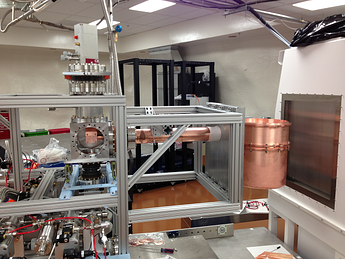
Postdoctoral Research Associate
Majorana Collaboration
University of North Carolina - Chapel Hill
Superconducting QuBits
Yale
While these qubits have many possible physical implementations, we make them out of superconducting circuits consisting for a non-linear inductance made from a Josephson Junction and capacitance. The circuits are fabricated with Aluminium on sapphire and to work, need to be cooled to dilution refridgerator temperatures of about 10mK. This not only makes the devices superconducting but also makes these circuits behave quantum mechanically.
Graduate Student, QuLab
Dept. of Applied Physics, Yale University
Ion Penning Traps
University of Granada
Department of Atomic Molecular and Nuclear Physics
University of Granada
The Atacama Cosmology Telescope
University of Pennsylvania
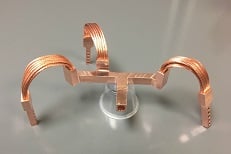
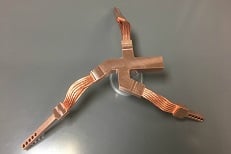
University of Pennsylvania